Ultrafast Molecular Dynamics (Molecular Movies)
The focus of this project is to understand and control, on a fundamental level, how light is converted to chemical energy and heat at the single-molecule level. Molecules convert light to chemical energy through conformational changes and to heat through vibrations on ultrafast time scales. Our work aims to capture this changes with atomic resolution, making a molecular movie where the motion of the atoms during a reaction can be observed. These observations are essential for gaining a solid understanding of the reaction mechanism and for building accurate models. The tool we use for this is ultrafast electron diffraction (UED). In this technique, a femtosecond laser pulse is used to excite the target molecules and trigger a reaction, and a femtosecond electron pulse is used to capture the molecular structure at a specific time during the reaction. The time-delay between the trigger laser pulse and the probe electron pulse can be varied very precisely, thus capturing snapshots of the structure at different times. To learn more about the UED read the instrument development section where we describe our contributions to the development of this technique.
We have demonstrated that UED can be used to capture the motion of atoms in isolated molecules, and have successfully captured previously inaccessible dynamics. We have observed the intrinsic quantum mechanical behavior of molecules, such as the dispersion and splitting of a nuclear wavepacket, we have determined the structure of short-lived intermediate states and observed coherent motions that persists after the molecule returns to the electronic ground state. During a dissociation reaction, we have captured the moment when the wavepacket splits and the reaction follows two different pathways at the same time, one in which a bond breaks and one in which it doesn’t. We are now working to apply UED to more complex reactions with the goal of developing general rules to create a new understanding of the dynamics of photo-excited molecules.
Our work in the news:
- (o-nitrophenol molecule) Nebraska today press release - Husker physicist part of team that captures groundbreaking 'molecular movie'. This work was also featured on the cover of the jounal.
- (CF3I molecule) Nebraska today press release - 'Molecular movie' captures chemical reaction on atomic scale. This work was also emphasized via the news on Phys.org.
- (Iodine molecule) Nebraska today press release - Heart of the matter: Physicists record movement of atomic nuclei. This work was also emphasized via the news on SLAC National Accelerator Laboratory, and featured as an Editor's suggestion and as an APS Physics.
Some references can be found below:
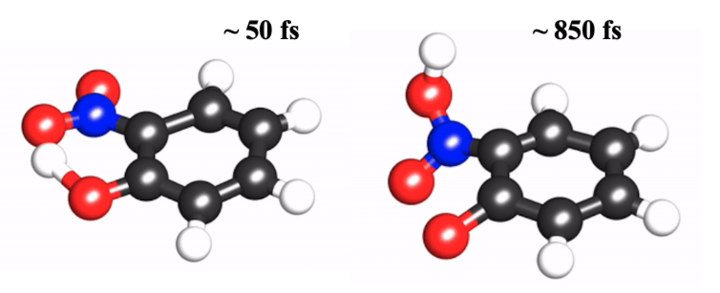
O-nitrophenol at ~50 fs and ~850 fs showing the different molecular structure. Its interesting dynamics and full molecular movie can be found here.
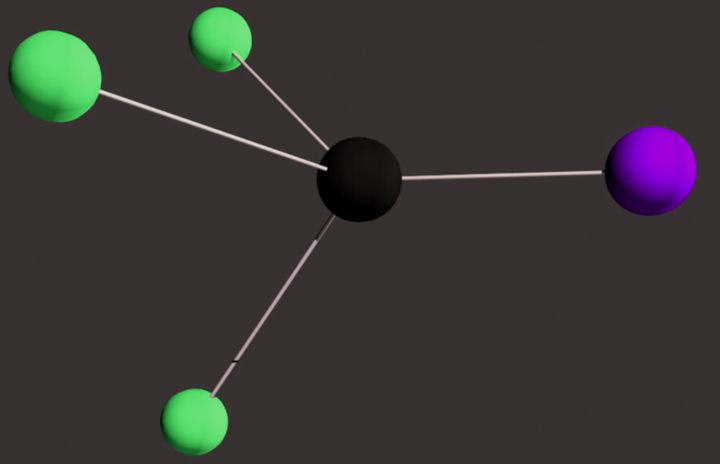
CF3I molecule with fluorine atoms (green), carbon (black), and Iodine (purple). Full video and information of molecular dynamics can be found here. Image is taken from Stanford University / SLAC National Accelerator Laboratory.
Three-dimensional Molecular Imaging
The goal of this project is to capture atomically resolved three-dimensional images of isolated molecules. Having access to accurate molecular structures is essential for understanding how they function. The majority of known molecular structures have been determined using either x-ray diffraction for crystallized molecules or NMR spectroscopy for molecules in solution. Recently, intense X-ray pulses have been used to study the structure of isolated molecules, but this method has not yet reached the required sub-Angstrom resolution to distinguish individual atoms. Gas electron diffraction, on the other hand, has been successful to determine structures of isolated molecules with very high (picometer) precision. However, this method has been limited in the size of molecules that can be studied due to the random orientation of the molecules in the gas phase. This averaging over orientations reduces the information content of the measurement and makes it more difficult to retrieve the molecular structure from the diffraction measurements.
In this project, we use a femtosecond laser pulse to align the molecules, and then record the diffraction pattern while the molecules are aligned. The laser pulse provides a rotational impulse to the molecules through an induced dipole interaction. The molecules start to rotate and become aligned after interacting with the laser pulse. This timing is important because at the time when the molecules are aligned, the laser pulse is gone and thus the aligned molecules can be studied free of any field that might distort their structure. A short electron pulse scatters from the molecules while they are aligned, and the diffraction pattern is recorded. We were able to demonstrate with this method the first three-dimensional images of isolated molecules with atomic resolution. This work involves optimizing the alignment process and developing new theoretical tools to simulate the alignment process and to retrieve structures from diffraction patterns. The work now continues to apply the method to larger and more complex molecules, and to move towards the direction of capturing 3D movies of molecular reactions by combining alignment and photoexcitation.
Some of the references can be found below:
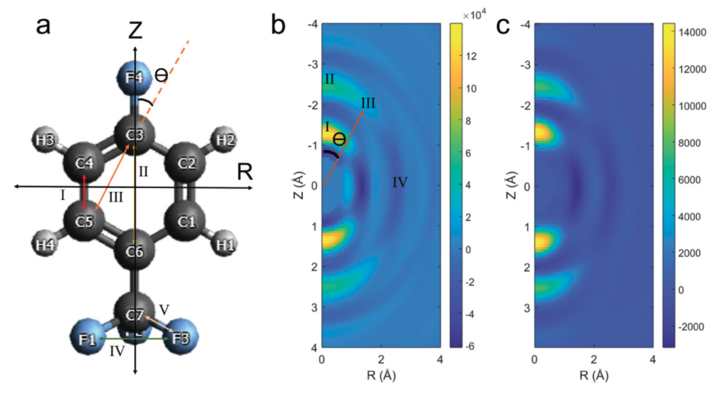
Our results on 4-flourobenzotriflouride. (a) Theoretical molecular structure. (b) Experimental diffraction. (c) simulated diffraction. The results highlight the approach of constructing 3D molecular structure from the anisotropic diffracted signal.
Instrument development
UED background
We have dedicated significant effort to developing the tools to perform ultrafast electron diffraction (UED) from isolated molecules (gas phase) experiments that will allow us to reach the required spatiotemporal resolution for three-dimensional imaging and to capture dynamics. A UED experiment comprises a short laser pulse, short electron pulse and a molecular beam with the target molecules. Femtosecond laser pulses have now become widely available and can be produced using commercial laser systems. The challenge today is in generating sufficiently bright and short electron pulses and synchronizing them with the laser pulses. In order to capture molecular dynamics that take place in femtosecond timescales, the electron pulses should have a correspondingly short duration. In addition, it is essential to be able to synchronize the laser and electron pulses equally well to preserve the temporal resolution. Bright electron pulses (with a high number of electrons in each pulse) are needed to provide sufficient scattering signal from diffuse gas targets. The standard setup for gas phase UED relies on a photocathode to generate electron pulses using a UV laser pulse. The laser pulse is split off from the same pulse that excites the molecules, which allows for synchronization of the pump laser and probe electron pulses. The photo-emitted electron pulses have an initial duration similar to that of the laser, however, the pulse duration rapidly broadens due to Coulomb repulsion between the electrons in the bunch. In standard UED setups the pulse broadening was controlled by minimizing the propagation distance and reducing the number of electrons per bunch to just a few thousands, making experiments very challenging due to the low scattering signals. Recently, there has been a rapid improvement in the technology required to make this experiments possible and we have been working to apply them to gas phase UED experiments. Below we describe some of the technological improvements that have pushed the field forward significantly.
More information on UED experiment can be found with these two review papers:
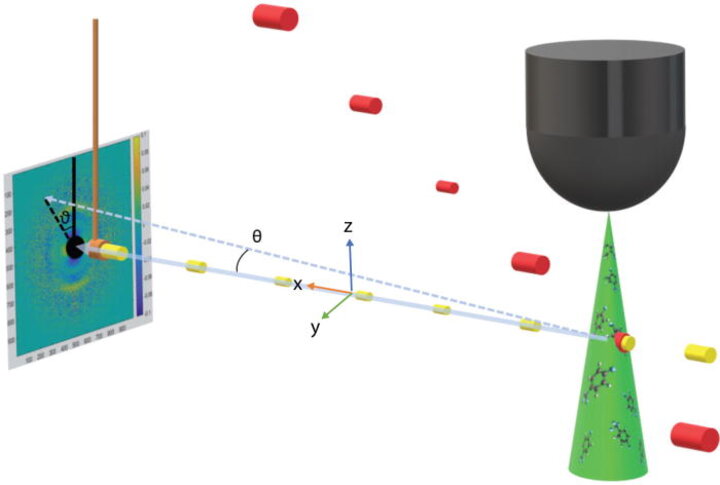
The UED experimental setup in our lab. The interaction region represented as the overlap between electron beam (yellow), laser (red), and the gas sample (green). Image taken from here.
Table-top gas phase UED
We have constructed a table-top setup to deliver femtosecond electron pulses on a gas target. This setup uses existing technology to compress electron bunches using an RF cavity. In this setup, a large number of electrons, on the order of a hundred thousand, are generated by shining a laser pulses on a photocathode. These electrons are accelerated in a DC field to an energy of 100 keV. The electron bunch rapidly broadens due to the Coulomb force and develops a velocity chirp (fast electrons in front, slow electrons in back). The pulse then enters a resonant radio frequency (RF) cavity that has a time dependent longitudinal electric field. The field is timed very precisely such that it slows down the leading electrons and speeds up the electrons in the back, reversing the velocity distribution and thus causing the electron pulse to compress at a predetermined position (the gas target). The main challenge in this type of instrument is to synchronize the cavity fields with the laser pulses, which can be done through feedback loops and careful control of the lab conditions. With this method, we have shown that we can deliver pulses with less than 200 fs duration on the target. In addition, we need to compensate for the different velocities of the electron and laser pulses as they traverse the sample, which leads to broadening of the time resolution. We have done this by tilting the pulse front of the laser and adjusting the angle between the beams to match the longitudinal velocity of the laser pulse to that of the electrons (about half the speed of light). This setup runs at a high repetition rate of 1-5 kHz which results in a high beam current. We are currently using this setup to perform imaging and dynamics experiments.
More about this topic can be found below:
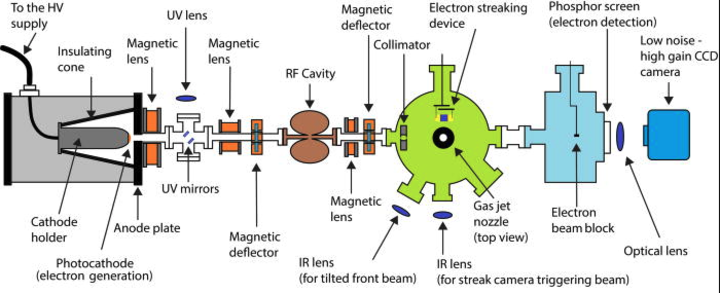
The design of our table-top UED. Thanks to all of our group members.
UED with Relativistic electron pulses at SLAC
We have, in collaboration with the UED group at SLAC National Accelerator Laboratory, constructed and implemented an experimental end station to perform gas phase UED experiments using an existing MeV photoelectron gun. In this setup, we take advantage of the fact that the electron beam is accelerated to relativistic energies using an RF accelerator to an energy of a few MeV. After reaching relativistic speeds, the problem of velocity mismatch becomes negligible, and the Coulomb broadening is significantly reduced due to relativistic effects. This setup is capable of delivering femtosecond pulses on target without the need to use additional pulse compression. After several years of development staring in 2015, the instrument has now operates as part of the LCLS user facility, making this technology more widely available. Many groups have taken advantage of this setup to carry out experiments. We are still involved in developing and improving the setup and regularly perform experiments at SLAC National Lab.
Some references can be found below:
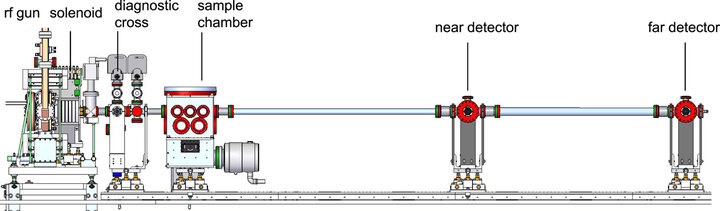
Schematic of MeV-UED beam line at SLAC. Image taken from here.
UED from liquid phase samples at SLAC National Lab.
Our group has participated in the development of a new instrument at SLAC National Lab to take advantage of the relativistic (MeV) electron gun to perform ultrafast electron diffraction (UED) experiments from samples in the liquid phase. This setup involves a new target chamber and a liquid jet with nanometer thickness. Samples can now be studied in solution in addition to in the gas phase. This will enable new science such as studying catalytic processes and comparing the dynamics of isolated molecules with that of molecules in solution. The setup has been demonstrated and first experiments carried out in liquid water and iodine in solution.
Some references can be found below:
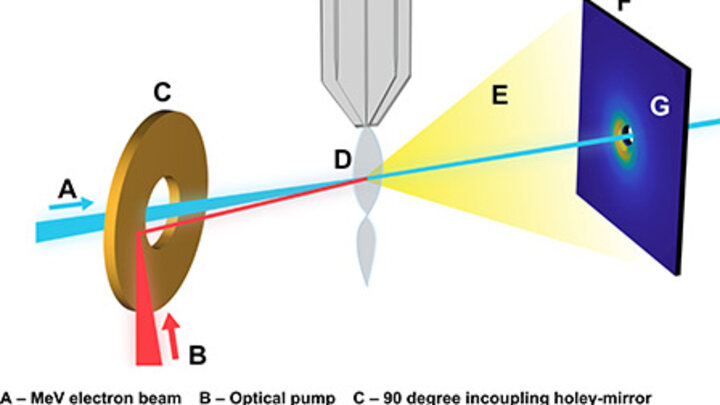
The design of UED for liquid-phase sample. Image taken from here.
Condensed matter UED.
We have recently modified our instrument such that we can now carry out experiments in solids and gas phase samples using the same instrument. The setup is interchangeable between solid and gas targets by swapping the gas manifold for a sample holder.
More on this topic can be found here.
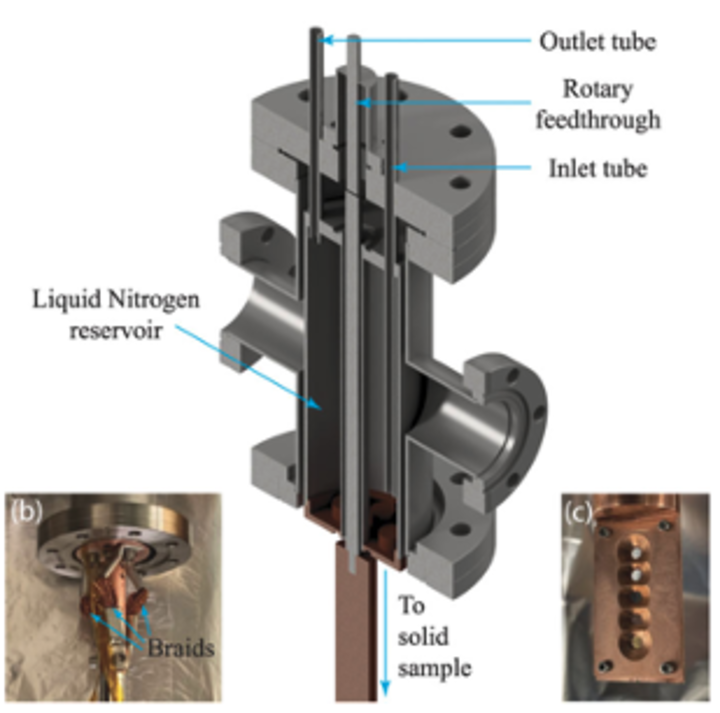
The design of the nozzle made by Dr. Yibo (former member), Prof. Centurion, and our colleagues. Image taken from Rev. Sci. Instrum. 94, 053001 (2023).
Mass-selected ion–molecule cluster beam apparatus.
This technique is for ultrafast photofragmentation studies. We have developed an instrument which produces a beam of anions to study photo-induced dissociative electron attachment experiments.
More on this topic can be found here.
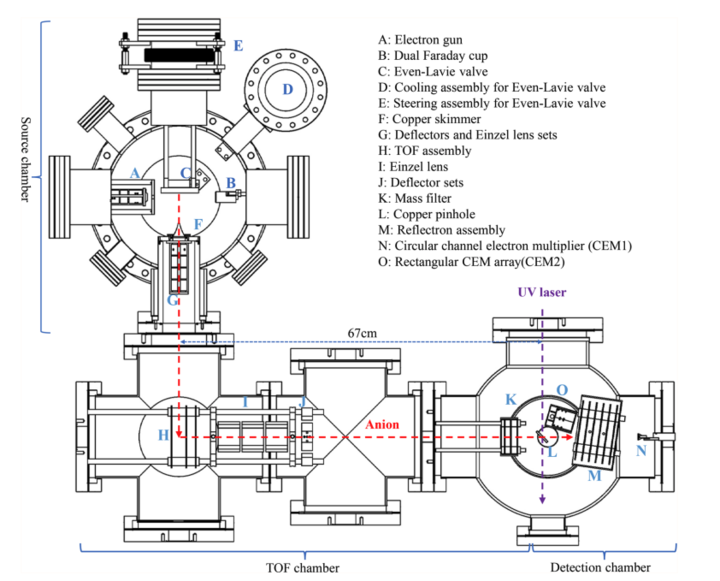
The design of the instrument made by Dr. Xiaojun (former member), Prof. Centurion, and our colleagues. Image taken from Rev. Sci. Instrum. 94, 095111 (2023).